Comparison of the Antibacterial Effect of Different Biological Silver Nanoparticles Synthetized and Integrated with Honeys
DOI:
https://doi.org/10.55121/nefm.v1i1.46Keywords:
Bee, Nanosilver, Green synthesis, Bactericidal, HoneysAbstract
This study compares the morphologies, zeta potentials, and antibacterial effects a total 12 different microcompounds containing honey and silver nanoparticles, in a novel study of the difference between honey samples in nanoparticle synthesis, as well as the antibacterial interaction that those honey samples can have with the silver nanoparticles synthetized using them. Microcompounds were synthetized by combining silver nitrate solution with a honey sample and performing one of methods of biogenic synthesis: sunlight exposure, basification to pH 5 or basification to pH 10. Samples of each microcompound were also submitted to heat treatment, obtaining thus heated variants. Morphology and size data were obtained by Dynamic Light Scattering (DLS) analysis and Scanning Electron Microscopy (SEM); while zeta potential was measured by Electrophoretic Light Scattering. Broth microdilution, time-kill curves and SEM were used to access the antibacterial effect. Mean diameter of particles inside all microcompounds varied between 100 nm and 150 nm; and the zeta potential varied depending on the honey used. Minimal Inhibitory Concentrations (MIC) of microcompounds were between 15 μM and 500 μM. Time-kill curves showed that microcompounds had a faster and stronger effect against Escherichia coli than Staphylococcus aureus. Microcompounds obtained by basification to pH 5 or by sunlight were bactericidal, as they were capable of inhibiting bacterial growth (resulting in an antibacterial efficiency of 100% in 24 hours) at 125 μM against S. aureus and 62.5 μM against E. coli. SEM micrographs showed bacterial cells with lower cell density, blebs and other alteration after microcompound treatment.
References
[1] O’Neill, J., 2020. Tackling drug-resistant infections globally: final report and recommendations. Review on Antimicrobial Resistance website. May 19, 2016. Available from: http://www.pewinternet.org/Presen-tations/2009/40-The-rise-of-the-e-patient.aspx. (Accessed July 13, 2020)
[2] European Centre for Disease prevention and Control, 2017. Antimicrobial resistance surveillance in Europe 2015. Annual Report Of The European Antimicrobial Resistance Surveillance Network (EARS-Net). Stockholm: ECDC.
[3] World Health Organization, 2017. Prioritization of pathogens to guide discovery, research and development of new antibiotics for drug-resistant bacterial infections, including tuberculosis. License WHO/ EMP/IAU/2017.12. Available from: https://apps.who. int/iris/handle/10665/311820?locale-attribute=pt&. (Accessed September 25, 2020)
[4] Interagency Coordination Group on Antimicrobial Resistance, 2019. No time to wait: Securing the future from drug-resistant infections. World Health Organization website. Available from: https://www. who.int/publications/i/item/no-time-to-wait-securing-the-future-from-drug-resistant-infections. (Accessed September 25, 2020)
[5] The Pew Charitable Trusts, 2016. A scientific roadmap for antibiotic discovery. The Pew Research Charitable Trusts website. Available from:https://www.pewtrusts.org/-/media/assets/2016/05/ascientificroadmapforantibioticdiscovery.pdf. (Accessed September 25, 2020)
[6] Lemire, J., Harrison, J., Turner, R., 2013. Antimicrobial activity of metals: mechanisms, molecular targets and applications. Nature Reviews Microbiology. 11(6), 371-384. DOI:https://doi.org/10.1038/nrmicro3028
[7] Chernousova, S., Epple, M., 2012. Silver as Antibacterial Agent: Ion, Nanoparticle, and Metal. Angewandte Chemie International Edition. 52(6), 1636- 1653. DOI:https://doi.org/10.1002/anie.201205923
[8] Kim, J., Kuk, E., Yu, K., et al., 2007. Antimicrobial effects of silver nanoparticles. Nanomedicine. 3(1), 95-101. DOI:https://doi.org/10.1016/j.nano.2006.12.001
[9] Wang, R., Neoh, K., Kang, E., et al., 2014. AntifoulingAntifouling coating with controllable and sustained silver release for long‐term inhibition of infection and encrustation in urinary catheters. Journal of Biomedical Materials Research. 103(3), 519-528. DOI:https://doi.org/10.1002/jbm.b.33230
[10] Gibson, D.J., Yang, Q., Kerekes, D.T., et al., 2014. Medical Honey and Silver Dressings Do Not Interfere with Each Other’s Key Functional Attributes. Wounds. 26(11), 309-316.
[11] Gupta, A., Mumtaz, S., Li, C., et al., 2019. Combatting antibiotic-resistant bacteria using nanomaterials. Chemical Society Reviews. 48(2), 415-427. DOI:https://doi.org/10.1039/c7cs00748e
[12] Gupta, A., Landis, R., Rotello, V., 2016. Nanoparticle-based antimicrobials: Surface functionality is critical. F1000Res. 5, 364. DOI:https://doi.org/10.12688/f1000research.7595.1
[13] Neu, H., 1992. The crisis in antibiotic resistance. Science. 257(5073), 1064-1073. DOI:https://doi.org/10.1126/science.257.5073.1064
[14] Wang, L., Hu, C., Shao, L., 2017. The antimicrobial activity of nanoparticles: present situation and prospects for the future. International Journal of Nanomedicine. 12, 1227-1249. DOI:https://doi.org/10.2147/ijn.s121956
[15] Zhao, Y., Tian, Y., Cui, Y., et al., 2010. Small molecule-capped gold nanoparticles as potent antibacterial agents that target gram-negative bacteria. Journal of the American Chemical Society. 132(35), 12349- 12356. DOI:https://doi.org/10.1021/ja1028843
[16] Fortas, W., Djelad, A., Dib-Bellahouel, S., et al., 2022. Ionic liquid-functionalised AlPO-34 material prepared by ionothermal method for efficient antibacterial property against Escherichia coli. Materials Research Innovations. DOI:https://doi.org/10.1080/14328917.2022.2095840
[17] Zhou, Q., Wang, T., Wang, C., et al., 2020. Synthesis and characterization of silver nanoparticles-doped hydroxyapatite/alginate microparticles with promising cytocompatibility and antibacterial properties. Colloids and Surfaces A: Physicochemical and Engineering Aspects. 585, 124081. DOI:https://doi.org/10.1016/j.colsurfa.2019.124081
[18] Balasooriya, E., Jayasinghe, C., Jayawardena, U., et al., 2017. Honey mediated green synthesis of nanoparticles: New era of safe nanotechnology. Journal of Nanomaterials. 1-10. DOI:https://doi.org/10.1155/2017/5919836
[19] El-Desouky, T., Ammar, H., 2016. Honey mediated silver nanoparticles and their inhibitory effect on aflatoxins and ochratoxin A. Journal of Applied Pharmaceutical Science. 6(6), 83-90. DOI:https://doi.org/10.7324/japs.2016.60615
[20] González Fá, A., Juan, A., Di Nezio, M., 2016. Synthesis and characterization of silver nanoparticles prepared with honey: The role of carbohydrates. Analytical Letters. 50(5), 877-888. DOI:https://doi.org/10.1080/00032719.2016.1199558
[21] Mittal, A., Chisti, Y., Banerjee, U., 2013. Synthesis of metallic nanoparticles using plant extracts. Biotechnology Advances. 31(2), 346-356. DOI:https://doi.org/10.1016/j.biotechadv.2013.01.003
[22] González-Miret, M., Terrab, A., Hernanz, D., et al., 2005. Multivariate correlation between color and mineral composition of honeys and by their botanical origin. Journal of Agricultural and Food Chemistry. 53(7), 2574-2580. DOI:https://doi.org/10.1021/jf048207p
[23] Bar, H., Bhui, D., Sahoo, G., et al., 2009. Green synthesis of silver nanoparticles using seed extract of Jatropha curcas. Colloids and Surfaces A: Physicochemical and Engineering Aspects. 348(1-3), 212- 216. DOI:https://doi.org/10.1016/j.colsurfa.2009.07.021
[24] Olaitan, P.B., Adeleke, O.E., Ola, I.O., 2007. Honey: a reservoir for microorganisms and an inhibitory agent for microbes. African Health Sciences. 7(3), 159-165. DOI:https://doi.org/10.5555/afhs.2007.7.3.159
[25] Obot, I., Umoren, S., Johnson, A., 2013. Sunlight-mediated synthesis of silver nanoparticles using honey and its promising anticorrosion potentials for mild steel in acidic environments. JMES. 4(6), 1013-1018. https://www.academia.edu/6634621/ Sunlight-mediated_synthesis_of_silver_nanoparticles_using_honey_and_its_promising_anticorrosion_ potentials_for_mild_steel_in_acidic_environments. (Accessed September 25, 2020)
[26] Philip, D., 2009. Honey mediated green synthesis of gold nanoparticles. Spectrochimica Acta Part A: Molecular and Biomolecular Spectroscopy. 73(4), 650- 653. DOI:https://doi.org/10.1016/j.saa.2009.03.007
[27] Philip, D., 2010. Honey mediated green synthesis of silver nanoparticles. Spectrochimica Acta Part A: Molecular and Biomolecular Spectroscopy. 75(3), 1078-1081. DOI:https://doi.org/10.1016/j.saa.2009.12.058
[28] Madhu, G., Kumar, A., Nair, S., 2019. Sunlight-induced honey-mediated green synthesis of silver nanoparticles. AIP Conference Proceedings. 2162(1). DOI:https://doi.org/10.1063/1.5130311
[29] Kwakman, P., Velde, A., Boer, L., et al., 2010. How honey kills bacteria. FASEB Journal. 24(7), 2576- 2582. DOI:https://doi.org/10.1096/fj.09-150789
[30] Kwakman, P., Zaat, S., 2011. Antibacterial components of honey. IUBMB Life. 64(1), 48-55. DOI:https://doi.org/10.1002/iub.578
[31] Cortopassi-Laurino, M., Gelli, D., 1991. Pollen analysis, physico-chemical properties and antibacterial action of Brazilian honeys from Africanized honeybees (Apis mellifera L) and stingless bees. Apidologie (Celle). 22(1), 61-73. DOI:https://doi.org/10.1051/apido:19910108
[32] Poli, J., Guinoiseau, E., Luciani, A., et al., 2018. Investigating the antibacterial action of Corsican honeys on nosocomial and foodborne pathogens. Journal of Apicultural Research. 57(1), 186-194. DOI:https://doi.org/10.1080/00218839.2017.1412564
[33] Al-Mamary, M., Al-Meeri, A., Al-Habori, M., 2002. Antioxidant activities and total phenolics of different types of honey. Nutrition Research. 22(9), 1041- 1047. DOI:https://doi.org/10.1016/s0271-5317(02)00406-2
[34] Alves, R., Souza, B., Carvalho, C., et al., 2005. Cost of honey production: A proposal for africanized bees and meliponines. 1st ed. Cruz das Almas: Universidade Federal da Bahia/SEAGRI-BA.
[35] Bueno-Costa, F., Zambiazi, R., Bohmer, B., et al., 2016. Antibacterial and antioxidant activity of honeys from the state of Rio Grande do Sul, Brazil. LWT. 65, 333-340. DOI:https://doi.org/10.1016/j.lwt.2015.08.018
[36] Clébis, V., Nishio, E., Scandorieiro, S., et al., 2019. Antibacterial effect and clinical potential of honey collected from Scaptotrigona bipunctata Lepeletier (1836) and Africanized bees Apis mellifera Latreille and their mixture. Journal of Apicultural Research. 1-11. DOI:https://doi.org/10.1080/00218839.2019.1681118
[37] Cushnie, T., Lamb, A., 2006. Errata for “Antimicrobial activity of flavonoids”. International Journal of Antimicrobial Agents. 27(2), 181. DOI:https://doi.org/10.1016/j.ijantimicag.2005.12.002
[38] Nishio, E., Ribeiro, J., Oliveira, A., et al., 2016. Antibacterial synergic effect of honey from two stingless bees: Scaptotrigona bipunctata Lepeletier, 1836, and S. postica Latreille, 1807. Scientific Reports. 6(1). DOI:https://doi.org/10.1038/srep21641
[39] Adams, C., Manley-Harris, M., Molan, P., 2009. The origin of methylglyoxal in New Zealand manuka (Leptospermum scoparium) honey. Carbohydrate Research. 344(8), 1050-1053. DOI:https://doi.org/10.1016/j.carres.2009.03.020
[40] Molan, P., Betts, J., 2004. Clinical usage of honey as a wound dressing: an update. Journal of Wound Care. 13(9), 353-356. DOI:https://doi.org/10.12968/jowc.2004.13.9.26708
[41] Latimer, G., 2019. Official methods of analysis of AOAC International. 21st ed. Gaithersburg, Maryland: AOAC International.
[42] Priz, M., 2014. Influence of Different Parameters on Wet Synthesis of Silver. (bachelor’s thesis). Enschede, NL: University of Twente.
[43] Tagad, C., Dugasani, S., Aiyer, R., et al., 2013. Green synthesis of silver nanoparticles and their application for the development of optical fiber based hydrogen peroxide sensor. Sensors and Actuators B:Chemical. 183, 144-149. DOI:https://doi.org/10.1016/j.snb.2013.03.106
[44] Patel, K., Deshpande, M., Gujarati, V., et al., 2016. Effect of heating time duration on synthesis of colloidal silver nanoparticles. Advanced Materials. 1141, 14-18. DOI:https://doi.org/10.4028/www.scientific.net/ amr.1141.14
[45] Clinical & Laboratory Standards Institute (CLSI), 2018. Methods for dilution antimicrobial susceptibility tests for bacteria that grow aerobically. 11th ed. CLSI standard M07. Wayne, PA: Clinical and Laboratory Standards Institute.
[46] Clébis, V., Proni, E., Sarimento, J.P., et al., 2021. Antibacterial activity of silver microparticles encapsulated with honeys from Apis mellifera and Scaptotrigona bipunctata. Brazilian Journal Of Animal And Environmental Research. 4(1), 933-948. DOI:https://doi.org/10.34188/bjaerv4n1-076
[47] National Committee for Clinical Laboratory Standards (NCCLS), 1999. Methods for determining bactericidal activity of antimicrobial agents: Approved guideline. Wayne (Pennsylvania): NCCLS.
[48] El-Desouky, T., Ammar, H., 2016. Honey mediated silver nanoparticles and their inhibitory effect on aflatoxins and ochratoxin A. Journal of Pharmaceutical Sciences. 6(6), 83-90. DOI:https://doi.org/10.7324/japs.2016.60615
[49] Haiza, H., Azizan, A., Mohidin, A., et al., 2013. Green synthesis of silver nanoparticles using local honey. NH. 4, 87-98. DOI:https://doi.org/10.4028/www.scientific.net/nh.4.87
[50] Raveendran, P., Fu, J., Wallen, S., 2006. A simple and “green” method for the synthesis of Au, Ag, and Au–Ag alloy nanoparticles. Green Chemistry. 8(1), 34-38. DOI:https://doi.org/10.1039/b512540e
[51] Meshram, S., Bonde, S., Gupta, I., et al., 2013. Green synthesis of silver nanoparticles using white sugar. IET Nanobiotechnology. 7(1), 28-32. DOI:https://doi.org/10.1049/iet-nbt.2012.0002
[52] Catauro, M., Tranquillo, E., Dal Poggetto, G., et al., 2018. Influence of the heat treatment on the particles size and on the crystalline phase of TiO2 synthesized by the sol-gel method. Materials (Basel). 11(12), 2364. DOI:https://doi.org/10.3390/ma11122364
[53] Granbohm, H., Larismaa, J., Ali, S., et al., 2018. Control of the size of silver nanoparticles and release of silver in heat treated SiO2-Ag composite powders. Materials (Basel). 11(1), 80. DOI:https://doi.org/10.3390/ma11010080
[54] Jiang, X.C., Chen, W.M., Chen, C.Y., et al., 2011. Role of temperature in the growth of silver nanoparticles through a synergetic reduction approach. Nanoscale Research Letters. 6(1), 32. DOI:https://doi.org/10.1007/s11671-010-9780-1
[55] Barrett, K., Barman, S., Yuan, J., et al., 2019. Ganong’s Review Of Medical Physiology. 26th ed. New York, N.Y.: McGraw-Hill Education LLC.
[56] Radovic-Moreno, A., Lu, T., Puscasu, V., et al., 2012. Surface charge-switching polymeric nanoparticles for bacterial cell wall-targeted delivery of antibiotics. ACS Nano. 6(5), 4279-4287. DOI:https://doi.org/10.1021/nn3008383
[57] Zinni, Y., 2020. Types of microorganisms & optimum pH. Sciencing. https://sciencing.com/types-microor-ganisms-optimum-ph-8618232.html. (Accessed July 14, 2020)
[58] Qiao, Z., Yao, Y., Song, S., et al., 2019. Silver nanoparticles with pH induced surface charge switchable properties for antibacterial and antibiofilm applications. Journal of Materials Chemistry B. 7(5), 830- 840. DOI:https://doi.org/10.1039/c8tb02917b
[59] Abbaszadegan, A., Ghahramani, Y., Gholami, A., et al., 2015. The effect of charge at the surface of silver nanoparticles on antimicrobial activity against gram-positive and gram-negative bacteria: A preliminary study. Journal of Nanomaterials. 1-8. DOI:https://doi.org/10.1155/2015/720654
[60] Official Journal of the European Union, 2011. Commission recommendation on the definition of nanomaterial (text with EEA relevance). Retrieved from: https://eur-lex.europa.eu/eli/reco/2011/696/oj. (Acessed September 25, 2020)
[61] Food and Drug Administration, 2014. Considering whether an FDA-regulated product involves the application of nanotechnology. Retrieved from: https://www.fda.gov/regulatory-information/ search-fda-guidance-documents/considering-whether-fda-regulated-product-involves-application-nanotechnology. (Accessed September 25, 2020)
[62] Santamarina, J., Cho, G., 2004. Soil behaviour: The role of particle shape. Jardine R, Potts D, Higgins K, ed. Soil Advances In Geotechnical Engineering: The Skempton Conference. 1st ed. London: Thomas Telford. 604-617. https://www.icevirtuallibrary.com/doi/ abs/10.1680/aigev1.32644.0035. (Accessed September 25, 2020) DOI:https://doi.org/10.1680/aigev1.32644.0035
[63] Berrezueta, E., Cuervas-Mons, J., Rodríguez-Rey, Á., et al., 2019. Representativity of 2D Shape Parameters for Mineral Particles in Quantitative Petrography. Minerals. 9(12), 768. DOI:https://doi.org/10.3390/min9120768
[64] Helmlinger, J., Sengstock, C., Groß-Heitfeld, C., et al., 2016. Silver nanoparticles with different size and shape: equal cytotoxicity, but different antibacterial effects. RSC Advances. 6(22), 18490-18501. DOI:https://doi.org/10.1039/c5ra27836h
[65] Pal, S., Tak, Y., Song, J., 2007. Does the Antibacterial Activity of Silver Nanoparticles Depend on the Shape of the Nanoparticle? A Study of the Gram-Negative Bacterium Escherichia coli. Applied And Environmental Microbiology. 73(6), 1712-1720. DOI:https://doi.org/10.1128/aem.02218-06
[66] Cheon, J., Kim, S., Rhee, Y., et al., 2019. Shape-dependent antimicrobial activities of silver nanoparticles. International Journal of Nanomedicine. 14, 2773-2780. DOI:https://doi.org/10.2147/IJN.S196472
[67] Escárcega-González, C., Garza-Cervantes, J., Vazquez-Rodríguez, A., et al., 2018. In vivo antimicrobial activity of silver nanoparticles produced via a green chemistry synthesis using Acacia rigidula as a reducing and capping agent. International Journal of Nanomedicine. 13, 2349-2363. DOI:https://doi.org/10.2147/ijn.s160605
[68] Fayaz, A., Balaji, K., Girilal, M., et al., 2010. Biogenic synthesis of silver nanoparticles and their synergistic effect with antibiotics: a study against gram-positive and gram-negative bacteria. Nanomedicine: NBM. 6(1), 103-109. DOI:https://doi.org/10.1016/j.nano.2009.04.006
[69] Hajipour, M., Fromm, K., Akbar Ashkarran, A., et al., 2012. Antibacterial properties of nanoparticles. Trends Biotechnol. 30(10), 499-511. DOI:https://doi.org/10.1016/j.tibtech.2012.06.004
[70] Khan, A., 2012. Medicine at nanoscale: a new horizon. International Journal of Nanomedicine. 7, 2997- 2998. DOI:https://doi.org/10.2147/IJN.S33238
[71] Khan, S., Mukherjee, A., Chandrasekaran, N., 2011. Studies on interaction of colloidal silver nanoparticles (SNPs) with five different bacterial species. Colloids and Surfaces B-Biointerfaces. 87(1), 129-138. DOI:https://doi.org/10.1016/j.colsurfb.2011.05.012
[72] Leid, J., Ditto, A., Knapp, A., et al., 2011. In vitro antimicrobial studies of silver carbene complexes: activity of free and nanoparticle carbene formulations against clinical isolates of pathogenic bacteria. Journal of Antimicrobial Chemotherapy. 67(1), 138- 148. DOI:https://doi.org/10.1093/jac/dkr408
[73] Morones, J., Elechiguerra, J., Camacho, A., et al., 2005. The bactericidal effect of silver nanoparticles. Nanotechnology. 16(10), 2346-2353. DOI:https://doi.org/10.1088/0957-4484/16/10/059
[74] Qais, F., Shafiq, A., Khan, H., et al., 2019. Antibacterial effect of silver nanoparticles synthesized using Murraya koenigii (L.) against multidrug-resistant pathogens. Bioinorganic Chemistry and Applications. 1-11. DOI:https://doi.org/10.1155/2019/4649506
[75] Sinha, R., Karan, R., Sinha, A., et al., 2011. Interaction and nanotoxic effect of ZnO and Ag nanoparticles on mesophilic and halophilic bacterial cells. Bioresource Technology. 102(2), 1516-1520. DOI:https://doi.org/10.1016/j.biortech.2010.07.117
[76] Sreelakshmi, C., Datta, K., Yadav, J., et al., 2011. Honey Derivatized Au and Ag Nanoparticles and Evaluation of Its Antimicrobial Activity. Journal of Nanoscience and Nanotechnology. 11(8), 6995-7000. DOI:https://doi.org/10.1166/jnn.2011.4240
[77] Ashkarran, A., Ghavami, M., Aghaverdi, H., et al., 2012. Bacterial Effects and Protein Corona Evaluations: Crucial Ignored Factors in the Prediction of Bio-Efficacy of Various Forms of Silver Nanoparticles. Chemical Research in Toxicology. 25(6), 1231- 1242. DOI:https://doi.org/10.1021/tx300083s
[78] Brudzynski, K., 2020. A current perspective on hydrogen peroxide production in honey. A review. Food Chemistry. 332, 127229. DOI:https://doi.org/10.1016/j.foodchem.2020.127229
[79] Baek, Y., An, Y., 2011. Microbial toxicity of metal oxide nanoparticles (CuO, NiO, ZnO, and Sb2O3) to Escherichia coli, Bacillus subtilis, and Streptococcus aureus. Science of the Total Environment. 409(8), 1603-1608. DOI:https://doi.org/10.1016/j.scitotenv.2011.01.014
[80] Lagbas, A., Pelisco, J., Riego, V., 2016. Antibacterial activity of silver nano/microparticles in chitosan matrix prepared using Mangifera indica and Chrysophyllum cainito leaf extracts and its application in pineapple (Ananas comosus) polyester fabric. IJACS. 4(1), 7-13. DOI:https://doi.org/10.1016/j.colsurfb.2011.05.012
[81] Gupta, A., Mumtaz, S., Li, C., et al., 2019. Combatting antibiotic-resistant bacteria using nanomaterials. Chemical Society Reviews. 48(2), 415-427. DOI:https://doi.org/10.1039/c7cs00748e
[82] Jimenez, M., Beristain, C., Azuara, E., et al., 2016. Physicochemical and antioxidant properties of honey from Scaptotrigona mexicana bee. Journal of Apicultural Research. 55(2), 151-160. DOI:https://doi.org/10.1080/00218839.2016.1205294
[83] Rani, G., Narasinga Rao, B., Shamilli, M., et al., 2018. Combined effect of silver nanoparticles and honey in experimental wound healing process in rats. BioMed Research International. 29(15), 3074-3078. DOI:https://doi.org/10.4066/biomedicalresearch. 29- 18-898
[84] Mohamed, D.S., Abd El-Baky, R.M., Sandle, T., et al., 2020. Antimicrobial activity of silver-treated bacteria against other multi-drug resistant pathogens in their environment. Antibiotics (Basel). 9(4), 181. DOI:https://doi.org/10.3390/antibiotics9040181
[85] Loo, Y.Y., Rukayadi, Y., Nor-Khaizura, M.A., et al., 2018. In vitro antimicrobial activity of green synthesized silver nanoparticles against Selected Gram-negative foodborne Pathogens. Front Microbiol. 9, 1555. DOI:https://doi.org/10.3389/fmicb.2018.01555
[86] Das B et al., 2017. Green synthetized silver nanoparticles destroy multidrug resistant bacteria via reactive oxygen species mediated membrane damage. Arabian Journal of Chemistry. 10(6), 862-876. DOI:https://doi.org/10.1016/j.arabjc.2015.08.008
[87] Abdelsattar, A.S., Hakim, T.A., Rezk, N., et al., 2022. Green synthesis of silver nanoparticles using Ocimum basilicum L. and Hibiscus sabdariffa L. extracts and their antibacterial activity in combination with phage ZCSE6 and sensing properties. Journal of Inorganic and Organometallic Polymers. 32, 1951- 1965. DOI:https://doi.org/10.1007/s10904-022-02234-y
[88] Amin, M., et al., 2014. Green synthesis of silver nanoparticles: Strucutural features and in vivo and in vitro therapeutic effects against Heliobacter pylori induced gastritis. Bioinorganic Chemistry and Applications. 4, 1-11. DOI:https://doi.org/10.1155/2014/135824
[89] Cushnie, T., O’Driscoll, N., Lamb, A., 2016. Morphological and ultrastructural changes in bacterial cells as an indicator of antibacterial mechanism of action. Cellular And Molecular Life Sciences. 73(23), 4471-4492. DOI:https://doi.org/10.1007/s00018-016-2302-2
[90] Klainer, A.S., Perkins, R.L., 1972. Surface manifestations of antibiotic-induce alterations in protein synthesis in bacterial cells. Antimicrob Agents Chemother. 1(2), 164-170. DOI:https://doi.org/10.1128/aac.1.2.164
[91] Thammawithan, S., Siritongsuk, P., Nasompag, S., et al., 2021. A Biological Study of Anisotropic Silver Nanoparticles and Their Antimicrobial Application for Topical Use. Journal of Veterinary Science. 8(9), 177. DOI:https://doi.org/10.3390/vetsci8090177
[92] Boberek, J., Stach, J., Good, L., 2010. Genetic evidence for inhibition of bacterial division protein FtsZ by berberine. PLoS One. 5(10), e13745. DOI:https://doi.org/10.1371/journal.pone.0013745
[93] Radman, M., 1975. SOS repair hypothesis: phenomenology of an inducible DNA repair which is accompanied by mutagenesis. Basic Life Sciences. 5A, 355-367. DOI:https://doi.org/10.1007/978-1-4684-2895-7_48
[94] Spratt, B.G., 1975. Distinct penicillin binding proteins involved in the division, elongation, and shape of Escherichia coli K12. Proceedings of the National Academy of Sciences of the United States of America. 72(8), 2999-3003. DOI:https://doi.org/10.1073/pnas.72.8.2999
[95] Toyofuku, M., Nomura, N., Eberl, L., 2019. Types and origins of bacterial membrane vesicles. Nature Reviews Microbiology. 17(1), 13-24. DOI:https://doi.org/10.1038/s41579-018-0112-2
[96] Lee, W., Kim, K.J., Lee, D.G., 2014. A novel mechanism for the antibacterial effect of silver nanoparticles on Escherichia coli. Biometals. 27(6), 1191- 1201. DOI:https://doi.org/10.1007/s10534-014-9782-z
[97] Dwyer, D.J., Camacho, D.M., Kohanski, M.A., et al., 2012. Antibiotic-induced bacterial cell death exhibits physiological and biochemical hallmarks of apoptosis. Molecular Cell. 46(5), 561-572. DOI:https://doi.org/10.1016/j.molcel.2012.04.02723
[98] Rai, M., Yadav, A., Gade, A., 2009. Silver nanoparticles as a new generation of antimicrobials. Biotechnology Advances. 27(1), 76-83. DOI:https://doi.org/10.1016/j.biotechadv.2008.09.002.
[99] Li, W.R., Xie, X.B., Shi, Q.S., et al., 2010. Antibacterial activity and mechanism of silver nanoparticles on Escherichia coli. Applied Microbiology and Biotechnology. 85(4), 1115-1122. DOI:https://doi.org/10.1007/s00253-009-2159-5
[100] Brudzynski, K., Sjaarda, C., 2014. Antibacterial compounds of Canadian honeys target bacterial cell wall inducing phenotype changes, growth inhibition and cell lysis that resemble action of β-lactam antibiotics. PLoS One. 9(9), e106967. DOI:https://doi.org/10.1371/journal.pone.0106967
[101] Elliott, T.S., Rodgers, F.G., 1985. Morphological response and growth characteristics of Legionella pneumophila exposed to ampicillin and erythromycin. Journal of Medical Microbiology. 19(3), 383- 390. DOI:https://doi.org/10.1099/00222615-19-3-383
[102] Tsang, K.W., Ng, P., Ho, P.L., et al., 2003. Effects of erythromycin on Pseudomonas aeruginosa adherence to collagen and morphology in vitro. European Respiratory Journal. 21(3), 401-406. DOI:https://doi.org/10.1183/09031936.03.00050903
[103] Burdett, I.D., Murray, R.G., 1974. Septum formation in Escherichia coli: characterization of septal structure and the effects of antibiotics on cell division. Journal of Bacteriology. 119(1), 303-324. DOI:https://doi.org/10.1128/JB.119.1.303-324.1974
[104] Siddique, M., Aslam, B., Imran, M., et al., 2020. Effect of Silver Nanoparticles on Biofilm Formation and EPS Production of Multidrug-Resistant Klebsiella pneumoniae. Biomed Research International. 1-9. DOI:https://doi.org/10.1155/2020/6398165
[105] Sadeghi-Aliabadi, H., Hamzeh, J., Mirian, M., 2015. Investigation of Astragalus honey and propolis extract’s cytotoxic effect on two human cancer cell lines and their oncogen and proapoptotic gene expression profiles. Advanced NanoBiomed Research. 4(42). DOI:https://doi.org/10.4103/2277-9175.151251
[106] Milić, M., Leitinger, G., Pavičić, I., et al., 2015. Cellular uptake and toxicity effects of silver nanoparticles in mammalian kidney cells. Journal of Applied Toxicology. 35(6), 581-592. DOI:https://doi.org/10.1002/jat.3081
[107] Akter, M., Sikder, M.T., Rahman, M.M., et al., 2017. A systematic review on silver nanoparticles-induced cytotoxicity: Physicochemical properties and perspectives. Journal of Advanced Research. 9, 1-16. DOI:https://doi.org/10.1016/j.jare.2017.10.008
[108] Albers, C.E., Hofstetter, W., Siebenrock, K.A., et al., 2013. In vitro cytotoxicity of silver nanoparticles on osteoblasts and osteoclasts at antibacterial concentrations. Nanotoxicology. 7(1), 30-36. DOI:https://doi.org/10.3109/17435390.2011.626538
[109] Gliga, A.R., Skoglund, S., Wallinder, I.O., et al., 2014. Size-dependent cytotoxicity of silver nanoparticles in human lung cells: the role of cellular uptake, agglomeration and Ag release. Part Fibre Toxicol. 11, 11. DOI:https://doi.org/10.1186/1743-8977-11-11
[110] Kaur, J., Tikoo, K., 2013. Evaluating cell specific cytotoxicity of differentially charged silver nanoparticles. Food And Chemical Toxicology. 51, 1-14. DOI:https://doi.org/10.1016/j.fct.2012.08.044
[111] Liu, P., Guan, R., Ye, X., et al., 2011. Toxicity of nano- and micro-sized silver particles in human hepatocyte cell line L02. Journal of Physics: Conference Series. 304, 012036. DOI:https://doi.org/10.1088/1742-6596/304/1/ 012036
[112] Jiao, Z.H., Li, M., Feng, Y.X., et al., 2014. Hormesis effects of silver nanoparticles at non-cytotoxic doses to human hepatoma cells. PLoS One. 9(7), e102564. DOI:https://doi.org/10.1371/journal.pone.0102564
[113] Lima, D.S., Gullon, B., Cardelle-Cobas, A., et al., 2017. Chitosan-based silver nanoparticles: A study of the antibacterial, antileishmanial and cytotoxic effects. Journal of Bioactive and Bompatible Polymers. 32(4), 397-410. DOI:https://doi.org/10.1177/0883911516681329
[114] Figueiredo, E.P., Ribeiro, J.M., Nishio, E.K., et al., 2019. New Approach For Simvastatin As An Anti-bacterial: Synergistic Effect With Bio-Synthesized Silver Nanoparticles Against Multidrug-Resistant Bacteria. International Journal of Nanomedicine. 14, 7975-7985. DOI:https://doi.org/10.2147/IJN.S211756
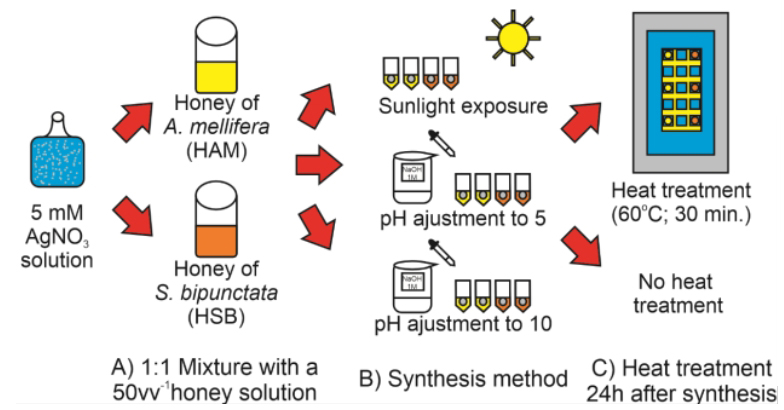
Downloads
How to Cite
Issue
Section
License
Copyright (c) 2022 Victor Hugo Clebis, Sara Scandorieiro, Wilma Aparecida Spinosa, Viviane Lopes Leite da Costa, Isabella Martins Lourenço, Amedea Barozzi Seabra, Renata Katsuko Katayama Kobayashi, Gerson Nakazato

This work is licensed under a Creative Commons Attribution 4.0 International License.